Types of Radioactive Decay
Navigation
- Introduction
- Nuclei stability
- Nuclear decay
- Alpha decay
- Beta decay
- Electron capture
- Internal conversion
- Decay chains
- Predicting type of decay
- References
Introduction
In the first part of the series, we explored the atomic nature of matter and the subatomic particles and forces within elements. In most natural cases, these atoms are stable and do not emit particles or energy over time. But what if an element is unstable? In this essay we explore how unstable nuclei work towards a stable configuration.
Nuclei stability
A spontaneous disintegration process known as radioactive decay (or nuclear decay) may occur whenever a nuclear can attain a more stable (i.e., more tightly bound) configuration by emitting radiation. Such non-stable nuclei may have an excess or deficiency of neutrons and can undergo a variety of transformational processes which may result in electromagnetic radiation, particles, or both.
All naturally-occurring nuclides with atomic numbers greater than 82 are radioactive and as such will emit radiation in their natural state. Uranium and thorium are radioactive elements with an extremely slow rate of decay, as we will discover later in the series.
Nuclear decay
Four useful principles for radioactive decay and nuclear reaction processes are as follows:
- Conservation of electric charge — implies that charges are neither created nor destroyed. Single positive and negative charges may, however, neutralize each other. It is also possible for a neutral particle to produce one charge of each sign.
- Conservation of mass number — does not allow a net change in the number of nucleons. However, the conversion of a proton to a neutron and vice versa is allowed.
- Conservation of mass and energy — implies that the total of the kinetic energy and the energy equivalent of the mass in a system must be conserved in all decays and reactions. Mass can be converted to energy and energy can be converted to mass, but the sum of mass and energy must be constant.
- Conservation of momentum — is responsible for the distribution of the available kinetic energy
among product nuclei, particles, and/or radiation. The total amount is the same before and after
the reaction even though it may be distributed differently among entirely different nuclides
and/or particles.
Alpha decay
Alpha decay or
KE represents the combined kinetic energy of the daughter nucleus (Thorium-230) and the
Beta decay
Beta decay or
Beta-minus decay (
Beta-plus decay (
Notice in the above equations there is a third type of type of particle, either the neutrino
Let’s pause to reflect: In beta-minus decay a neutron is converted to a proton and in beta-plus decay a proton becomes a neutron. In both cases the atom is attempting to find a more stable configuration—it stands to reason then that beta-minus decay will occur when there is an excess of neutrons and beta-plus when there is a deficiency.
Electron capture
Electron capture is a process that occurs when nuclei with a proton excess captures an electron from one of the inner orbits, typically the innermost orbit (or K-shell) and immediately combines the electron with a proton to form a neutron within the nucleus. This electron capture (EC) is sometimes called K-capture and a typical example of shown below:
To gain mass in this process would result in a violation of the conservation of energy, as the neutrino is carrying off some small amount of energy. A meaningful reduction in mass, then, will result in greater availability of energy which will appear as gamma radiation—a high-energy electromagnetic radiation that originates in the nucleus. In addition, x-rays will be given off when an electron from one of the higher energy shells moves to fill the vacancy in the K-shell. Figure 1 below illustrates this process:
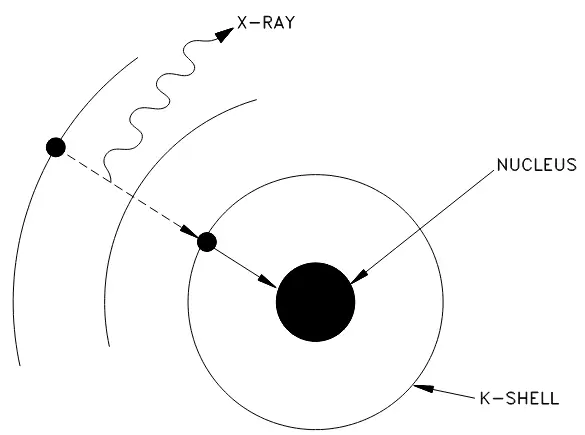
Figure 1: Orbital electron capture
A competing process known as positron emission results in the production of the same daughter product but has more stringent requirements—the mass of the daughter product must be less than the mass of the parent by an amount equal to at least twice the mass of an electron, in order to account for the positron ejected from the nucleus and the daughter product having one less orbital electron than the parent.
Internal conversion
Typically, an excited nucleus will move from an excited to ground state by way of gamma radiation. However, in some cases this gamma ray (photon) interacts with an innermost orbital electron and the energy of the photon is transferred to the electron. We say that the gamma ray has undergone internal conversion and that electron is ejected from the atom, with a kinetic energy equal to the gamma energy minus the binding energy of the orbital electron. This results in an orbital electron dropping to a lower energy state to effectively fill this gap, accompanied by x-ray emission as discussed above.
Decay chains
In the types of decays listed above, an unstable atoms is seeking stability but it is not necessarily the case that only one decay is required, especially for larger nuclides. A decay chain lists the original unstable nuclide, the steps in the decay and the final stable nuclide. As above, arrows represent the decays, decay types above the arrows and the half-life (explained later) below. Below is the decay chain for rubidium-91:
Predicting type of decay
Radioactive nuclides tend to decay in a predictable way, resulting in a daughter nuclide that lies closer to what is called the line of stability. Figure 2 below illustrates the type of decay that nuclides will typically undergo, depending on their atomic properties. Note: these are general rules and do have many exceptions, especially in the region of the heavy nuclides.

Figure 2: Types of radioactive decay relative to the line of stability
This makes sense intuitively—particles below and on the right of the line of stability have more neutrons than is stable and thus beta-minus decay will occur, particles above and to the left will reduce the excess protons by converting them to neutrons in beta-plus decay or electron capture (combining an electron with a proton to form a neutron).
References
- Department of Energy. (2015). DOE Fundamentals Handbook, Nuclear Physics and Reactor Theory, Volume 1 of 2 [Ebook]. Retrieved 28 December 2020, from https://www.standards.doe.gov/standards-documents/1000/1019-bhdbk-1993-v1.
- Shultis, J., & Faw, R. (2005). Fundamentals of nuclear science and engineering. CRC Press.